Regeneration
In order to reuse the sensor chip surface the analyte must be removed, but the ligand must stay intact. This so-called regeneration procedure has to be evaluated empirically because the combination of physical forces responsible for the binding are often unknown, and the regeneration conditions must not cause irreversible damage to the ligand (1), (2).
The most frequent regeneration method used, is to inject a low pH-buffer such as 10 mM Glycine pH 1.5–2.5 (2). This works probably because most proteins become partly unfolded and positively charged at low pH. The protein binding sites will repel each other and the unfolding will bring the molecules further apart (2).
Other procedures use high pH, high salt or specific chemicals to break the interaction. It is important to choose the mildest regeneration conditions that completely dissociate the complex. In addition, other molecules can be added such as antibodies, ligand and ligand analogues that bind to the analyte to diminish rebinding of the analyte during dissociation.
Type of bond | Acidic | Basic | Hydrophobic | Ionic |
Strength | ||||
Weak | pH > 2.5 | pH < 9 | pH < 9 | |
10 mM glycine/HCl | 10 mM HEPES/NaOH | 25–50 % ethylene glycol | 0.5–1 M NaCl | |
1–10 mM HCl | 0.02% SDS | |||
0.5 M formic acid | ||||
Intermediate | pH 2-2.5 | pH 9-10 | pH 9-10 | |
0.5 M formic acid | 10–100 mM NaOH | 50 % ethylene glycol | 1–2 M MgCl2 | |
10 mM Glycine/HCl | 10 mM Glycine/NaOH | 0.5–0.5% SDS | 1–2 M NaCl | |
10 mM Glycine/HCl | ||||
0.85% H3PO4 | ||||
Strong | pH < 2 | pH > 10 | pH > 10 | |
1 M formic acid | 50–100 mM NaOH | 25-50% ethylene glycol | 2–4 M MgCl2 | |
10–100 mM HCl | 6 M guanidinechloride | 0.5% SDS | ||
10–50 mM Glycine/HCl | 1 M ethanolamine | |||
0.85% H3PO4 | ||||
0.1% trifluoracetic acid |
Some regeneration solution have undesired effects on the ligand or sensor chip. Regeneration solutions can cause:
- loss of ligand function. The ligand is not biological active anymore after a strong regeneration solution. Always start with a mild solution and short contact times.
- persistent non-specific binding, incomplete regeneration and memory effects. Regeneration solution can induce irreversible changes in ligand and analyte. In the first case some additional non-specific binding may be observed and in the second some residual analyte may remain on the surface.
- matrix effects, i.e. changes in extension of the dextran matrix on the sensor chip surface because of changes in the pH or ionic strength. The effect of these variations is usually small in terms of absolute response, but may be significant to experiments where highest sensitivity is required. Matrix effects may have time constants ranging from seconds (for e.g. small changes in pH or ionic strength) to minutes or even hours (larger changes as for example regeneration with extreme conditions). Matrix effects are normally directly related to the mass concentration of the immobilized ligand, with greater effects at higher concentrations.
- conformational changes in the immobilized ligand resulting from changes in buffer composition. These changes may resemble matrix effects, but are caused by changes in the ligand rather than in the dextran matrix.
- relatively slow matrix effects in the ligand surface, which can cause baseline drift at the beginning of each cycle. Introduce a stabilization time at the beginning of the sensorgram to allow baseline stabilization if this is a problem (3).
Finding the best regeneration condition
The main idea of the cocktail regeneration method (4), (2), (5) is to target several binding forces simultaneously by mixing different chemicals. By using several chemicals in one cocktail, it is likely to disturb the binding at less harsh conditions preserving the ligand.
Andersson (2) presented on the BIA-Symposium 1998 a nice poster about regeneration and a method to find relatively quick and easy the best regeneration conditions. On his poster, he presented six stock solutions from which he started to mix new complex regeneration solutions.
Acidic | Equal volumes of oxalic acid, H3PO4, formic acid and malonic acid, each in 0.15 M, mixed and adjusted to pH 5.0 with NaOH. |
Basic | Equal volumes of ethanolamine, Na3PO4, piperazin and glycine each in 0.20 M, mixed and adjusted to pH 9.0 with HCl. |
Ionic | A solution of KSCN (0.46 M), MgCl2(1.83 M), urea (0.92 M), guanidin-HCl (1.83 M). |
Non polar water soluble solvents | Equal volumes of DMSO, formamide, ethanol, acetonnitril and 1-butanol were mixed. |
Detergents | A solution of 0.3% (w/w) CHAPS, 0.3% (w/w) zwittergent 3-12, 0.3% (v/v) tween 80, 0.3% (v/v) tween 20 and 0.3% (v/v/) triton X-100. |
Chelating | A 20 mM EDTA solution. |
The first step is to mix new regeneration solutions from the basic stock solutions. Every cocktail consists of three parts — either three different or one and two parts water. After injection of the analyte, the first regeneration solution is injected. The effect is measured and given in a percentage of regeneration (0–100 %). If the regeneration was below 10%, the next regeneration solution is injected. If the regeneration is more than 50%, then new analyte is injected. This process is repeated until all solutions have been tested.
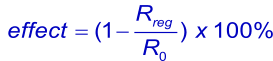
Determine from the best three regeneration solutions what they have in common. Choose three stock solutions with the best regeneration and mix new regeneration solutions. Again, inject analyte and regeneration solutions.
Repeat the procedure until a good regeneration solution is found.
References
(1) | BIACORE ABBIACORE Getting Started. (1998). |
(2) | Andersson, K. et alExploring buffer space for molecular interactions. J.Mol.Recognit. 12: 310-315; (1999). |
(3) | BIACORE ABBIACORE Instrument Handbook. (1998). |
(4) | Andersson, K. et alIdentification and optimization of regeneration conditions for affinity- based biosensor assays. A multivariate cocktail approach. Analytical Chemistry 71: 2475-2481; (1999). |
(5) | BIACORE ABBIACORE Application Handbook. (1998). |